In the last few days I’ve had the privilege of giving short talks at a conference in Barcelona and to a morning seminar for an offshoot of the Technology Strategy Board in the UK. (Thank you very much to the Spanish ceramic products company ROCA for sponsoring the extremely illuminating Barcelona conversation. And to SNCF for making train travel from London to Barcelona so comfortable and efficient).
I wanted to make two central points in these presentations. First, driving down the costs of renewable technologies for electricity generation is going to get increasingly expensive. I suggest it might be better to invest more in R+D and less in subsidy payments for production. By 2020, the UK will be spending at least £7bn a year on direct payments to generators that own wind, solar and other low-carbon sources of power. R+D spending will be less than 5% of this. The balance isn't right.
Second, I wanted to pose a question that I think is being too often ignored: new Chinese nuclear power stations being built with Westinghouse AP1000 technology seem to be costing about $2,000 per kilowatt of power capability. And the US nuclear plants in the middle stages of construction, such as Vogtle 3, also seem very cheap compared to the astronomical sums now quoted for Finnish, French and British plants using Areva’s competing EPR technology. The proposed power station at Hinkley in Somerset is going to cost of the order of four times the price for the Vogtle unit. Why is the UK apparently willing to finance – through loan guarantees and high and fixed prices for electricity - a particular technology that increasingly looks outdated, over-complicated and very difficult to construct?
Let’s look at nuclear power first. The parts of the world that invested heavily in first and second generation nuclear power stations have seen extravagant increases in the construction cost of nuclear power. In France the price doubled between the first nuclear power station and the last. The cost of the new plant now under construction in Normandy is still escalating. But it’s a reasonable prediction that the cost will finally come in at around 6 times the cost (inflation-adjusted, of course) of the pioneer stations of the late 1970s.

If we had the figures for the still uncompleted Olkiluoto EPR reactor in Finland, the numbers would be similar to the Flamanville example. I thought it was amusing that in a recent announcement Areva blamed ‘Finnish lawyers’ for the delay and cost overruns. Finland has about the lowest numbers of lawyers per capita in the EU, Britain has the highest. If Areva cannot cope with Finnish lawyers, we know they are going to be rapidly overwhelmed by their far more numerous and bloodthirsty UK equivalents.
The systematic inflation in nuclear power extends to the US. There most estimates see average nuclear power construction costs more than tripling since the 1960s to around 80% of the projected Flamanville cost. (Please note that the US numbers are in dollars, the French figures in euros).

The consistent and rapid inflation in nuclear costs has dulled observers into an unthinking pessimism. Every time I mention Olkiluoto, eyes roll and experienced commentators simply say that the EPR has added unnecessary complexity in an efforts to demonstrate 100% safety. All the savings from the technological advances of the last sixty years in nuclear power stations have been taken back in the form of enhanced safety features.
(Actually, though this not strictly relevant, it’s worth mentioning that the pioneers of the nuclear power industry in the UK thought it would be possible to make electricity for about 2.5 pence per kWh when writing in 1956.[1] This is about 55p in modern money, or six times what EDF is promised for power produced at Hinkley. So things have actually got better in some ways since the first nuclear power plant was opened at Calder Hall in the mid-fifties).
Does modern nuclear energy have to cost so much? The Chinese example should give us pause. The first new generation plants will be completed this year or early in 2015. Of course we don’t know whether the numbers are strictly comparable, but the cost appears to be about $2,000 per kilowatt of generating capacity. At today’s exchange rate, this means that the new Chinese power stations will cost a quarter of Flamanville’s price. And the Chinese are expecting future plants to come in at 80% of the first reactors. Why aren’t people flying off to Beijing to find out exactly why this is happening? Let’s put this in a UK context. If we could copy China and build nuclear at $1,600 a kilowatt, the cost of switching to low carbon electricity would fall from perhaps £6,000 a household to little more than £1,000. Shouldn’t we be trying to copy Chinese engineers?
As usual, one gets quite a lot of half-baked semi-racism when one asking this question. You hear that the Chinese aren’t sufficiently concerned about safety, either of employees or local people. Or that the Chinese are using forced labour. And so on.
But some people also notice that the reactor design in China is different and, second, that the country’s extraordinary amount of high quality civil engineering in the last decade has also given it unparalleled knowledge of how to pour concrete and forge steel cheaply and well. If I had a role in UK energy policy I would be asking Chinese companies to come here and build nuclear power stations for us. And trying very hard to work out very quickly whether the AP 1000 design is safe and why it appears to be so much cheaper than the EPR model.
The construction of AP 1000 reactors at Vogtle in US state of Georgia started later than the Chinese reactors. So we may not really know what the real cost is. However the plant’s owners are saying that the cost is likely to be $3,100 a kilowatt. That’s nearly twice the Chinese cost but it’s still a huge potential saving over the costs in Finland, France and, in prospect, Britain. Please can we hear from a well-qualified engineer as to why this might so?

Just to make the obvious point, let me stress that the reason that construction costs of nuclear are so important is that the running expenditures are so low. If we can cut the capital costs of new power station, this improvement feeds into dramatic price reductions. Copying the suggested cost of Vogtle in the UK would hugely reduce the subsidy needed for nuclear electricity. My guess is that instead of £92.50 per megawatt at Hinkley we need to offer a price of less than £45 for a reactor built like Vogtle. Chinese costs would reduce the required price even further to well below the costs of fossil fuel power. And, as should be apparent to everybody, if we don’t get low-carbon costs below fossil fuels, we are never going to maintain a global push for decarbonisation. Electorates simply won’t stand for it.
This leads on to the second question I tried to look at my talks. Is it right to drive cost reductions in renewable technologies by use of direct production subsidies that are adding increasing amounts to domestic bills? Or should we be spending more, much more, on fundamental research and development? The argument is this. Broadly speaking, we can achieve cost improvements in any technology either by accumulating production experience (usually called ‘the learning curve’) or by targeting improvements in technology. It is often difficult to disentangle the two phenomena but I still think the distinction is useful. Put another way, should we trying to cut prices by ‘learning by doing’ or by ‘learning by research’?
Governments around the world have backed away from energy research. In the 1970’s administrations that had been frightened by the OPEC oil embargo put big sums into R&D, particularly into nuclear but also into wind. Outside France, that investment largely failed, and failed catastrophically. Energy R&D then plummeted around the world. A decade ago, UK energy research was costing just a few tens of millions a year. (It has gone up somewhat since).

Instead of research, governments decided to back ‘learning by doing’. They offered production subsidies (now often called Feed In Tariffs) to get investors to put capital into wind, solar and a few other technologies. This, they correctly foresaw, would allow manufacturers and installers to cut costs. The learning curve (which I pedantically call the ‘experience curve’) swung into action as it almost always does (except in nuclear). As the accumulated volumes of wind turbines that had been built doubled, costs fell by about 14%. The rate of learning for PV looks greater, at about 20%.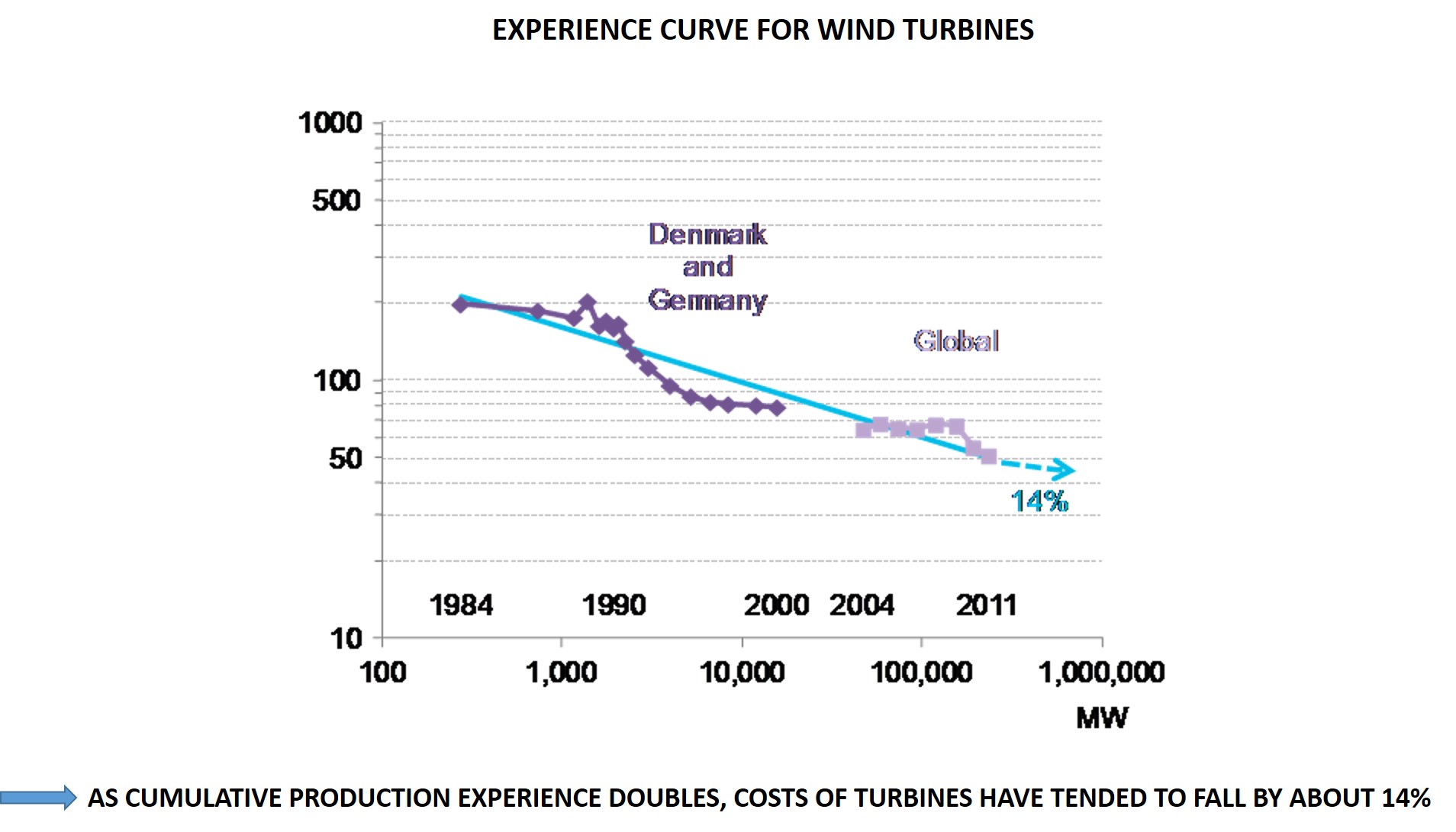
When I talk about the experience curve, I don’t just mean the cost improvements arising from larger turbines, or bigger factories. For some almost magical reason, costs fall in a reasonably consistent and predictable way just because companies get better at making the turbine. It’s obvious why governments like Feed In Tariffs. Prices do go down without any obvious reason.
Compare this with ‘learning by research’. Put $100m into some crazy new idea for making solar panels and you are 95% likely to fail. Faced with media always eager to locate apparent stupidity, or even corruption, no government minister or senior official will want to back the latest idea coming out of the Oxford Science Park or an automotive supplier in Swindon knowing that she is fairly certain to look really foolish within a year. As a result of bureaucratic risk aversion, direct subsidies are going to cost the UK consumer £3bn this year while government energy research languishes at perhaps 6% of this figure. (To be honest, this estimate is almost guess – nowhere can I find an authoritative estimated of the total budget for government energy R+D is for 2014/15, The DECC documents I have seen are extraordinarily confusing and obfuscatory).
There’s an even more important problem as well. At present the UK government doles out its R&D budget in tiny spoonfuls. It gives £1m to this nascent technology, a few hundred thousand to another, and a generous £3m to a particular favourite. In my view this not just pointless, it is actively counter-productive. Little dollops of cash have a truly awful effect.
I’ll try to explain why this is. Engineers leaving universities or companies with a brilliant idea need money. And government will often provide this, even when venture capital does not. Bodies like the grandiosely named Technology Strategy Board will drip small amounts of cash into many ideas-based companies. It won’t actually be enough to pay for real innovation or commercialisation but it will be just about enough to keep the business alive.
Why is this bad? It means that the talented engineer will stay beavering in his lab night after night hoping to make marginal improvements that can justify the next request for government rations. He works for the government, not for the marketplace. Actually, it would be far better if he failed, went broke and returned to the labour market where he could exercise his (undoubtedly real) skills on another project.
Spreading a hundred million pounds or so a year over perhaps two hundred potential innovators in the UK energy market is a mistake. It would be far better to gamble (and, to be absolutely clear, this is gambling) tens of millions on the technologies that might really make a difference. This is the way it would happen in the States but even there the disastrous experience of backing PV venture Solyndra has chilled the willingness to try to back winners.
But back winners we must, however unfashionable this task seems to be. Without large punts, progress on cost reduction in renewable technologies will slow.
Let’s look at one example of this. What will the iron law of the experience curve do to the cost of wind turbines over the next few years? More precisely, if we do decide to continue to back wind globally, but only by means of production subsidy, we’re reliant on the expected magical cost reduction of 14% for every doubling of accumulated (not yearly) production.
Let’s say we want to cut the cost of wind in half to make it competitive with fossil fuels across the world. If the 14% experience curve continues to operate, we’ll need to expand total accumulated production about eighteen fold. I guess that the world now has about 250 gigawatts of production experience of turbine production. That would mean we’d need 4,500 gigawatts of wind turbines to get down to 50% of the current cost. And this amount is equivalent to almost the entire world generating capacity today.

It’s also going to be very expensive indeed. To cut costs by 14% when the world had made 1 gigawatt of wind turbines required another1 gigawatt to be manufactured. To do so now requires 250 gigawatts to come off the production line. And although the subsidy needed has fallen considerably since the days of 1 gigawatt accumulated production, it has probably only declined by five or six fold per unit of capacity. So overall subsidy costs might be fifty times as much.
Of course this is a very generalised argument. In some windy places, including much of the central USA, wind is probably already almost competitive with new gas-fired plants. In other countries, wind will never be a real choice. I just want to make the point that for governments to rely on the experience or learning curve to drive down costs inevitably becomes more and more expensive. By contrast, sponsoring R&D doesn’t cost more as technology advances. It probably costs less.
So my case is simply that whether it be wind, PV, anaerobic digestion, heat pumps, geothermal energy, tidal lagoons or micro-hydro, genuine background R&D must make more and more sense. Intelligently directed in large amounts per idea, it may create large improvements in costs.
I know of three technologies (in wind, PV and AD) that may have the potential to reduce the underlying price of energy by at least 50%. None will come to market through the aid of Feed In Tariffs. All of them need tens of millions of pounds, which may well not be available from commercial sources. A small fraction of the billions now used to subsidise existing technologies needs to be diverted into directly backing companies like these.
But, of course, this is unlikely to happen. The renewable industries, who are so ready to criticise fossil fuel subsidies, are now addicted to their own guaranteed cash streams from government and have growing lobbying power. The genuine innovation that we need is in danger of never happening.
[1] This figure is taken from Calder Hall by Kenneth Jay, Methuen, 1956.