The purpose of this draft paper is to assess what will happen if, as expected, many gigawatts of intermittent renewables are added to the UK grid alongside large amounts of standby gas power. I use actual data from spring 2013 to model what will happen in 2030 if the expected portfolio of low carbon sources of electricity is constructed. In particular, I try to estimate how much the back-up gas plants will be used and how much surplus and unusable electricity will be generated when the wind is blowing strongly or demand is relatively low.
I suggest that the two principal issues facing the UK grid will be using the huge seasonal surpluses of electricity arising in late spring, summer and early autumn and, second, how to finance the construction of tens of gigawatts of standby power which may be used less than 10% of the time.
I conclude by tentatively putting forward a view that the right way to deal with these issues may possibly be to invest even more heavily in renewables (and less in gas standby) and use the increased surpluses to produce methane for use in the gas grid. This may be a cheaper and more energy-secure solution than current proposals. It will also make decarbonisation of heat easier than currently expected.
(As always, I'm very grateful for comments on this article, however critical.)
Electricity demand and supply in 2030
Electricity supply.
a) Generation portfolio. With adjustments, I use the latest figures from the Committee on Climate Change (CCC). [1] The CCC says that ‘decarbonisation of the power sector is key to reducing emissions across the economy and would also enhance energy security’. It proposes four broadly similar scenarios that enable the UK to cut emissions from electricity generation to below 50 grams of CO2 per kilowatt hour. All these scenarios involve large amounts of nuclear power, wind generation, biomass burning, electricity production from fossil fuels using carbon capture and storage and unabated generation from conventional combined cycle gas plants (CCGT). I have merged these four possible future plans into a single estimate but simplified it to only include nuclear, wind, CCS, biomass, unabated gas and solar PV.[2]
The figures in table 1 are based on the following key assumptions
a) Nuclear. The government reaches agreement with the power and construction companies over the price that will be paid for electricity produced by nuclear power stations. As a result, two or three consortia built about 8 power stations by 2030.[3]
b) CCS. The government must also reach agreement over the price paid for power from CCS-equipped fossil fuel plants. As important, experiments and pre-commercial deployments need to happen quite quickly, if large plants are to be ready by 2030.
c) Wind. Offshore and onshore farms are projected to both contribute about 25 GW of capacity. The key issue is whether offshore wind costs decline at the rate expected by the CCC.
d) Biomass. Electricity generation from burning biomass is included in the government’s plans for 2020 and financial incentives are provided. I have assumed no expansion of biomass beyond the CCC’s figure for 2020.
e) CCGT. For the UK to have security of electricity supply, it needs to have enough ‘dispatchable’ generation capacity to meet peak winter needs. At the moment of likely maximum power requirements in early evenings in December and January, no PV is available and wind output may be negligible. So CCGT and nuclear, biomass and CCGT must cover the total requirement. In the last few years, the peak has been about 60 GW and I have suggested a figure of 40 GW of gas-fired power generation, leaving 20 GW to be provided by the other reliable power sources and a 5 GW safety margin. The CCC’s scenarios range between 40 GW and 46 GW.
Table 1
Central scenario for 2030 – generation capacity
Nuclear |
12 gigawatts (GW) |
CCS |
11 GW |
Wind |
50 GW |
Biomass |
4 GW |
CCGT |
40 GW |
Solar PV |
10 GW |
|
|
Total |
127 GW |
Electricity demand
In recent years electricity use has been gently falling. This change has been caused by improved energy efficiency and a weak economy. In addition, higher electricity prices have choked off demand.
The CCC assumes that electricity generation will rise from about 350 terawatt hours (TWh) in 2011 to between 403 and 465 TWh in 2030, an increase of between about 15 and 30 percent. The Committee isn’t clear in its most recent report why this increase will happen but previous publications from the CCC have pointed to the likelihood of increased electricity use from the use of heat pumps for domestic heating and from growth in the number of electric cars.
Other sources are less bullish about power demand. (Electricity demand is lower than electricity generation because of losses in the distribution system and use of electricity by the generators themselves). The most recent work by the National Grid suggests lower figures than those projected by the CCC.[4] National Grid’s three scenarios offer estimates of 2030 electricity demand that vary from a figure very similar to today’s level in its ‘Slow Progression’ scenario to estimates about 10% higher in its Gone Green forecast and 20% higher in the ‘Accelerated Growth’ view.
Projections for 2030
In the following section, I have estimated how much electricity is generated by the portfolio of generating plant in Table 1 during a portion of 2030. My approach was to assume that the daily pattern of spring demand in 2030 – rising from about 5 am to a plateau from 9am to 7pm and then falling sharply – is identical to 2013.
Figure 1 shows how electricity production varied for each of 4,300 half hour periods from February 23rd to May 23rd.
Figure 1
Electricity production, including imports, for half hour periods, expressed in megawatts
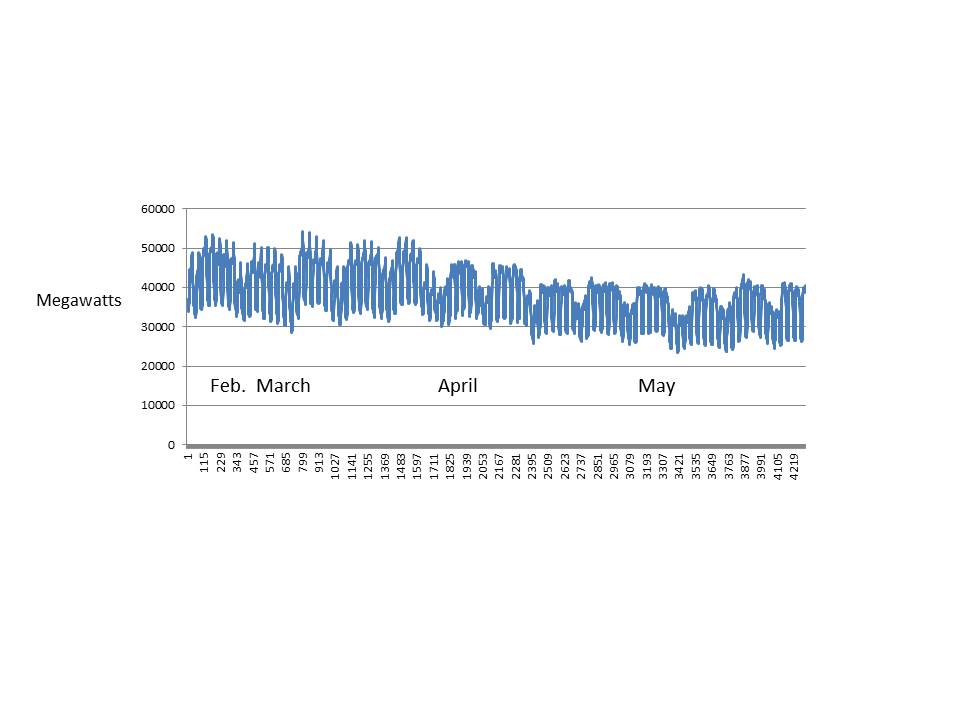
The chart shows the expected daily variation with production rising to a peak at the end of the working day/beginning of the evening and then falling to a much lower nighttime level. It also exhibits the weekly cycle of lower weekend and Bank Holiday power needs. Average demand levels were highest during March 2013 because of the unusually cold weather, peaking consistently at over 50,000 megawatts (50 GW). By May, peak demands averaged around 40 GW, with nightime levels falling to around 25 GW, down from 30-35 GW in March.
The next step was to estimate the source of electricity by type of generator in 2030. I have done this in the following way.
a) I used the 3 months electricity production data from late February to late May 2013. The manager of UK electricity trading arrangements, Elexon, publishes data for each half hour period. This information includes data on how much power is produced by nuclear, wind and all other types of generator.[5]
b) This 3 months of data provides estimates of the total amount of electricity generated by wind power in each half hour. For this 3 month period, the capacity of wind connected to the UK National Grid was 7.15 GW. The estimate in Table 1 is that this will rise to 50 GW by 2030. Therefore if the weather patterns in spring 2030 were to exactly the same as in spring 2013 we can plausibly assume that seven times as power will be generated. (7.15 GW multiplied by 7 equals about 50 GW, the assumption in Table 1 for the amount of wind capacity in 2030).
c) Nuclear power stations will operate continuously although some portion of capacity may be unavailable because of maintenance. I assume that 10 GW work continuously during the 2030 spring period.
d) CCS plants will also be working continuously. This is because they will be paid a standard and unchanging contract price for electricity. It will therefore make financial sense to operate them all the time.
e) The same is true for the 4 GW of biomass capacity.
f) I have assumed a standard daily profile for PV production, starting at daybreak and rising to midday and falling as the afternoon proceeds. This profile varies only by the month of operation with May output being much higher than production in late February. Average daily output figures for each month per kW of installed capacity are taken from records of a small rooftop PV installation.
g) Imports of electricity: in spring 2013, the UK imported significant quantities of power from France and the Netherlands. The 2030 forecasts assume no net imports. (This assumption is relaxed later in this paper).
To summarise: I have taken actual 2013 electricity generation for each half hour in a three month spring period and then used the predicted portfolio of generating capacity in 2030 to show the makeup of electricity production in that year. Nuclear, CCS and biomass plants (totalling an available figure of 25 GW, slightly less than maximum capacity because some plants will be undergoing maintenance) work continuously in 2030. Production from wind turbines is seven times greater than the 2013 actual figures for wind generation. Solar PV generates electricity during the daytimes according to a set pattern. CCGT plants operate when the amount of wind and PV generation would be insufficient to bridge the gap between the dispatchable power sources (nuclear, CCS and biomass) and the total 2013 demand levels.
The results
Overall
25 GW of generating plant that is working continuously will generate almost enough power to cover the minimum needs of a night in late spring. By contrast, peak demands of 55 GW in the early evening of the last days of February will require either substantial wind-generated electricity or the use of some of the 40 GW of CCGT plants.
When the wind is blowing strongly, the UK is likely to have a substantial surplus of power. By contrast, quiet days will mean continuous use of gas-fired generation.
In the three months under study, UK generators attached to the main distribution network and adding in imports produced about 81.2 terawatt hours (TWh) of electricity (or 81,200 gigawatt hours). In 2030, the portfolio of predicted generating plant working under the same conditions would produce 94.5 terawatt hours. This would be made up as follows
Table 2
Makeup of spring 2030 electricity production if demand conditions and wind speeds the same as 2013
Nuclear |
23.6 TWh |
Wind |
32.0 TWh |
CCS |
21.5 TWh |
PV |
2.4 TWh |
Biomass |
8.6 TWh |
|
|
Total before CCGT |
88.1 TWh |
|
|
CCGT |
6.4 TWh |
|
|
Total including CCGT |
94.5 TWh |
|
|
Surplus over electricity generation need |
13.4 TWh (about 16%) |
This is the key result: with the portfolio identified by the CCC, and the same demand pattern in 2030 as in spring 2013, CCGT plants need to generate 6.4 TWh and, second, the variability of wind means that the UK nevertheless produces 13.4 TWh too much electricity. This surplus must be exported, stored or dumped because electricity demand must match electricity supply every minute of every year. Figure 2 shows when the UK is in surplus, and by how much, over the 4,300 half hour periods under study.
Figure 2
UK net electricity surplus (-ve) or deficit (+ve) before taking in account the use of CCGT plants, expressed in megawatts

Several features of this chart stand out.
a) As winter ends, surpluses of electricity become more common. From mid-April onwards, periods of deficit, and hence need to back up intermittent wind using CCGT, become much more rare.
b) Surpluses and deficits can be very large in any one period. Deficits peaked during March at levels over 20 GW. But deficits of this size tend to be very short-lived. The peak deficit of around 25 GW at point 903 was followed 5 hours later by a need for only 8 GW of back-up gas generation.
c) Surpluses in the coldest months (such as around points 700 and 1400) are driven by major storms. These events last for several days and can produce sustained and very large surpluses. The importance of these sustained surpluses will be discussed later.
d) The April/May surpluses are only interrupted by very short periods of need for CCGT back-up. The implications of this will also be mentioned in the section on storage.
Demand for gas generation
One criticism of the approach in this paper is to say that the CCC assumed higher overall electricity demand in 2030. (In future work I will examine the implications of greater aggregate demand). The calculations here suggest that 40 GW of CCGT back-up will only provide about 6.4 terawatt hours of power in the late February – late March 2030 season. This is equal to only 7.5% of potential output. The CCC itself admits that the gas back-up stations will be used for ‘less than 20%’ of their capacity.[6]
An important question is whether the late February/late May 2013 electricity generation figures are reasonably typical. Multiplied up to the entire year, the figures under study suggest a total electricity generation of about 330 terawatt hours, just less than a quarter of actual total annual need for this year. In other words, the period I used has average daily electricity needs of very slightly below the mean for the year. But the variance is only about 5% and would not significantly affect the results in this paper: the total yearly demand for gas back-up generation will probably mean that CCGT plants stand idle for a very large portion of the time.
The CCC estimates that CCGT stations cost about £600 per kilowatt of power. The public policy question is therefore whether 40 GW of back-up stations are worth the £24bn that they are likely to cost (and for which electricity users will have to pay). If the late February/late May 2013 period is typical, these power stations will only provide about 8% of the total electricity production of the UK in 2030.
Storage
Swings in wind power production and variations in daily demand mean that electricity storage will become increasingly important. However, the results of my analysis suggest that conventional energy storage technologies are not particularly helpful in assisting management of electricity deficit and surplus. The reason? Periods of surplus, usually created by windy periods of a couple of days, generate far more electricity than could conceivably be stored using conventional technologies.
To validate this last assertion, I modelled the addition of 50 gigawatt hours of storage to the electricity network. The current storage capacity for UK electricity, almost entirely in the form of hydro-electric power plants that pump water to a high reservoir when demand is low and let it flow downhill when electricity is scarce, is only about 10 gigawatt hours. 50 GWh is a five times expansion of this capacity, which will also be created largely from new ‘pumped storage’ reservoirs, principally in the Scottish Highlands.[7] But even 50 GWh makes very little difference, particularly when the UK in 2030 will often have days or weeks of consistent surplus in the warmer, lighter six months of the year.
My simple model suggests that the periods of electricity surplus in the three months under study created a total excess of around 13.4 terawatt hours. Less than 10% of that (1.1 TWh) could be stored and regenerated as electricity during times of deficit in the hours and days after the surplus was created.
Even huge scale expansion of conventional storage, costing billions of pounds, doesn’t solve the fundamental problem that electricity surpluses and deficits will not principally be diurnal, balancing out during the course of day, but multi-day (in the case of typical Atlantic storms in winter) or, more importantly, seasonal. With the pattern of electricity generation capacity proposed by the CCC, the UK will be in deficit in winter and in sustained surplus during the summer. 50 GWh is about 6% of the average daily electricity demand of the UK and therefore incapable of being a significant contributor to electricity supply. To put this more vividly, mid-April 2013 saw consistent winds that would have resulted in a total surplus of 4.0 TWh over a five day period (more than 1% of total UK annual electricity need). 50 GWh (0.05 TWh) of storage reservoirs would have reused little more than 1% of this.
Exporting the surplus
Instead of storing the electricity abundance during windy weather, the UK could export the surplus to countries linked to the National Grid through interconnectors. (At the moment, the UK is typically a net importer of power from France and other places). Interconnectors have a limited capacity to take current. Presently, the links to France, Netherlands and Ireland have a total size of about 3 GW.
I modelled creating export capacity of 5 GW, 8 GW and 10 GW for surplus UK electricity. I did this by looking at each half hour period in the three month study period and when there was a surplus calculating whether it could be carried abroad on interconnectors of the three sizes. The results are in the table below.
Table 3
How much of the 13.4 TWh hour surpus in late February/late May could be exported?
Interconnector capacity |
5 GW |
8 GW |
10 GW |
|
|
|
|
Exported |
5.2 TWh |
7.5 TWh |
8.9 TWh |
Remaining surplus |
8.2 TWh |
5.8 TWh |
4.5 TWh |
|
|
|
|
Total surplus |
13.4 TWh |
13.4 TWh |
13.4 TWh |
This analysis shows that a 10 GW interconnector could accept about two thirds of the surplus generated in the three month period. Even a 5 GW interconnector would be able to export over 40% of the excess. The problem remains that when the UK is in surplus, the rest of Europe probably will be as well. High winds over the British Isles will mean excess in other countries close to the UK. As an illustration of this, we can look at the point of maximum wind power in the UK in the three month period under investigation. At around 3.30pm on 22nd March, turbines were delivering 5.3 GW to the electricity grid. In Germany, the peak was at almost the same time.[8] On that occasion Spain saw a daily peak of 12 GW an hour earlier than the UK.[9]
At times when the UK has surplus electricity, the rest of Europe – also heavily and increasingly reliant on wind power – will generally also be wishing to export. Although it may be possible to transmit the excess, the price of wholesale electricity will fall to zero or below. (Electricity can trade for negative prices if producers are paid for their production through subsidies such as feed in tariffs). Building bigger and bigger interconnectors to other European countries is not the solution to the oversupply problem.
The conclusions of this paper
This paper uses actual data from spring 2013 and modified CCC forecasts for the portfolio of generating plants to project the pattern of electricity demand and supply in 2030. It shows that the requirement that electricity demand is always met implies that the UK will have to have up to 40 GW of standby CCGT plants. If the UK acquires 50 GW of wind power (a seven fold increase on today but the CCC regards the figure as achievable) then the average gas plant will be used about 10% of the time. Expensive assets will lie unused for months on end but will have to be paid for by electricity users.
As importantly, a strong portfolio of nuclear and CCS plants will mean that baseload needs are met at periods of very low demand, such as summer weekends. 50 GW of wind power will mean about 40% of electricity demand is met from wind but much of this electricity will be – in effect – wasted because it cannot be stored and exports will have no value.
If the weather conditions of 2013 are replicated in 2030, the three month period of late February to late May will result in a surplus of about 13.4 TWh in the period. Extrapolation to a full year is difficult but might be as much as 40-50 TWh, or perhaps 15% of total electricity demand.
The following question arises. The CCC says that by 2030 all low carbon technologies, including CCS (and unabated gas) will be (very roughly) at the same cost.[10] Is the rational national strategy to choose 50 GW and some PV and expect substantial amounts of dumped electricity? Or will it be better to invest in ‘Power to Gas’ the only conceivable way of storing energy seasonally?[11] [12]
Demand for heat for homes and other buildings is a larger part of the UK’s total energy requirements than is electricity. It is also varies far more seasonally. Is the right route forward to hugely over-invest in renewable electricity sources, such as PV, and then convert the surplus on a sunny July day into methane for use in December? It seems to me that if the UK wants to decarbonise the entire economy, and not just electricity production, that this might well be the right way forward. The rise and rise of solar PV makes this more and more likely. Within two decades we are likely to see PV on a large portion of all roofs, domestic and other. This will mean, as already in Germany, that for four hours a day, six months a year net demand for grid electricity will fall substantially below current levels. If export is unavailable, then storage of power as methane may be economically attractive.
I will try to explore these topics in a further paper.
[1] Next steps on Electricity Market Reform – securing the benefits of low-carbon investment, Committee on Climate Change, May 2013
[2] For reasons that are very unclear, the CCC almost ignores PV in its 2030 projections. However, recent investment interest has resulted in rapid expansion of large PV farms using ground mounted panels. See, for example, http://www.larkenergy.co.uk/news/uk-s-largest-solar-farm-completed-and-grid-connected/. I project that falling costs of PV installations will take the UK’s PV capacity up from about 3 GW at the end of 2013 to 10 GW in 2030. For comparison, Germany already had about 34 GW in May 2013
[3] These assumptions are distilled from Next steps on Electricity Market Reform – securing the benefits of low-carbon investment, pages 30 and 31 and elsewhere in the CCC document.
[5] This information is published here: http://www.bmreports.com/bsp/bsp_home.htm . 3 months power production data is located by clicking on ‘Current/Historic’ underneath the ‘Generation by Fuel Type’ graph. These charts work best in Internet Explorer.
[6] Page 31 of the CCC’s Next steps on Electricity Market Reform – securing the benefits of low-carbon investment
[7] The utility SSE has planning permission to build a 30 GWh pumped storage plant at Coire Glas at a cost of £800m.
[8] The German figure is taken from the data produced by Dr Bruno Berger at the Fraunhofer Institute. (www.ise.fraunhofer.de)
[10] See Figure 1.3 in the CCC’s recent Next steps on Electricity Market Reform – securing the benefits of low-carbon investment
[11] Power to Gas refers to any process that takes electricity and uses it to generate hydrogen through electrolysis and then adds the hydrogen to CO2 to make methane, the principal ingredient of conventional natural gas.